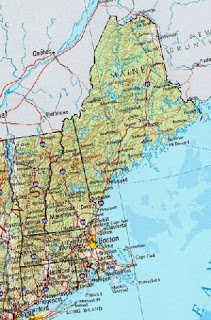
68 percent of New England and Mid-Atlantic beaches eroding
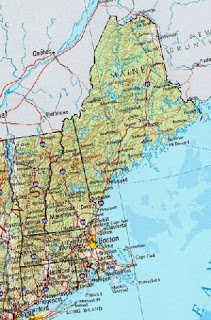
Scientists delve into ‘hotspot’ volcanoes along Pacific Ocean Seamount Trail
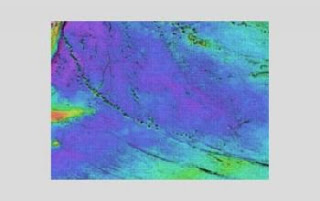
Geoscientists have just completed an expedition to a string of underwater volcanoes, or seamounts, in the Pacific Ocean known as the Louisville Seamount Trail.
There they collected samples of sediments, basalt lava flows and other volcanic eruption materials to piece together the history of this ancient trail of volcanoes.
“Finding out whether hotspots in Earth’s mantle are stationary or not will lead to new knowledge about the basic workings of our planet,” says Rodey Batiza, section head for marine geosciences in the National Science Foundation’s (NSF) Division of Ocean Sciences.
Tens of thousands of seamounts exist in the Pacific Ocean. Expedition scientists probed a handful of the most important of these underwater volcanoes.
Koppers led the expedition aboard the scientific research vessel JOIDES Resolution, along with co-chief scientist Toshitsugu Yamazaki from the Geological Survey of Japan at the National Institute of Advanced Industrial Science and Technology.
Over the last two months, scientists drilled 1,113 meters (3,651 feet) into the seafloor to recover 806 meters (2,644 feet) of volcanic rock.
“The sample recovery during this expedition was truly exceptional. I believe we broke the record for drilling igneous rock with a rotary core barrel,” says Yamazaki.
Igneous rock is rock formed through the cooling and solidification of magma or lava, while a rotary core barrel is a type of drilling tool used for penetrating hard rocks.
Trails of volcanoes found in the middle of tectonic plates, such as the Hawaii-Emperor and Louisville Seamount Trails, are believed to form from hotspots–plumes of hot material found deep within the Earth that supply a steady stream of heated rock.
As a tectonic plate drifts over a hotspot, new volcanoes are formed and old ones become extinct. Over time, a trail of volcanoes is formed. The Louisville Seamount Trail is some 4,300 kilometers (about 2,600 miles) long.
“Submarine volcanic trails like the Louisville Seamount Trail are unique because they record the direction and speed at which tectonic plates move,” says Koppers.
“The challenge,” says Koppers, “is that no one knows if hotspots are truly stationary or if they somehow wander over time. If they wander, then our calculations of plate direction and speed need to be re-evaluated.”
“More importantly,” he says, “the results of this expedition will give us a more accurate picture of the dynamic nature of the interior of the Earth on a planetary scale.”
Recent studies in Hawaii have shown that the Hawaii hotspot may have moved as much as 15 degrees latitude (about 1,600 kilometers or 1,000 miles) over a period of 30 million years.
“We want to know if the Louisville hotspot moved at the same time and in the same direction as the Hawaiian hotspot. Our models suggest that it’s the opposite, but we won’t really know until we analyze the samples from this expedition,” says Yamazaki.
In addition to the volcanic rock, the scientists also recovered sedimentary rocks that preserve shells and an ancient algal reef, typical of living conditions in a very shallow marine environment.
“We were really surprised to find only a thin layer of sediments on the tops of the seamounts, and only very few indications for the eruption of lava flows above sea level,” says Koppers.
The IODP Louisville Seamount Trail Expedition wasn’t solely focused on geology.
More than 60 samples from five seamounts were obtained for microbiology research.
Exploration of microbial communities under the seafloor, known as the “subseafloor biosphere,” is a rapidly developing field of research.
Using the Louisville samples, microbiologists will study both living microbial residents and those that were abundant over a large area, but now occupy only a few small areas.
They will examine population differences in microbes in the volcanic rock and overlying sediments, and in different kinds of lava flows.
They will also look for population patterns at various depths in the seafloor and compare them with seamounts of varying ages.
Samples from the Louisville Seamount Trail expedition will be analyzed to determine their age, composition and magnetic properties.
The information will be pieced together like a puzzle to create a story of the eruption history of the Louisville volcanoes.
Ground-based lasers vie with satellites to map Earth’s magnetic field
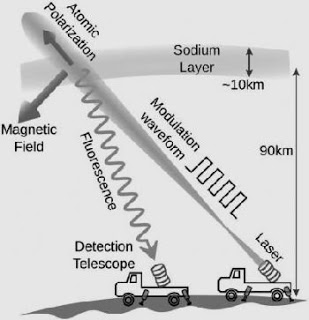
“Normally, the laser makes the sodium atom fluoresce,” said Dmitry Budker, UC Berkeley professor of physics. “But if you modulate the laser light, when the modulation frequency matches the spin precession of the sodium atoms, the brightness of the spot changes.”
Budker and three current and former members of his laboratory, as well as colleagues with the European Southern Observatory (ESO), lay out their technique in a paper appearing online this week in the journal Proceedings of the National Academy of Sciences.
Various satellites, ranging from the Geostationary Operational Environmental Satellites, or GOES, to an upcoming European mission called SWARM, carry instruments to measure the Earth’s magnetic field, providing data to companies searching for oil or minerals, climatologists tracking currents in the atmosphere and oceans, geophysicists studying the planet’s interior and scientists tracking space weather.
Ground-based measurements, however, can avoid several problems associated with satellites, Budker said. Because these spacecraft are moving at high speed, it’s not always possible to tell whether a fluctuation in the magnetic field strength is real or a result of the spacecraft having moved to a new location. Also, metal and electronic instruments aboard the craft can affect magnetic field measurements.
Laser guide stars
The idea was sparked by a discussion Budker had with a colleague about of the lasers used by many modern telescopes to remove the twinkle from stars caused by atmospheric disturbance. That technique, called laser guide star adaptive optics, employs lasers to excite sodium atoms deposited in the upper atmosphere by meteorites. Once excited, the atoms fluoresce, emitting light that mimics a real star.
Telescopes with such a laser guide star, including the Very Large Telescope in Chile and the Keck telescopes in Hawaii, adjust their “rubber mirrors” to cancel the laser guide star’s jiggle, and thus remove the jiggle for all nearby stars.
It is well known that these sodium atoms are affected by the Earth’s magnetic field. Budker, who specializes in extremely precise magnetic-field measurements, realized that you could easily determine the local magnetic field by exciting the atoms with a pulsed or modulated laser of the type used in guide stars. The method is based on the fact that the electron spin of each sodium atom precesses like a top in the presence of a magnetic field. Hitting the atom with light pulses at just the right frequency will cause the electrons to flip, affecting the way the atoms interact with light.
“It suddenly struck me that what we do in my lab with atomic magnetometers we can do with atoms freely floating in the sky,” he said.
Portable laser magnetometers
In practice, a 20- to 50-watt laser small enough to load on a truck or be attuned to the orange sodium line (589 nanometer wavelength) would shine polarized light into the 10 kilometer-thick sodium layer in the mesosphere, which is about 90 kilometers overhead. The frequency with which the laser light is modulated or pulsed would be shifted slightly around this wavelength to stimulate a spin flip.
The decrease or increase in brightness when the modulation is tuned to a “sweet spot” determined by the magnitude of the magnetic field could be as much as 10 percent of the typical fluorescence, Budker said. The spot itself would be too faint to see with the naked eye, but the brightness change could easily be measured by a small telescope.
“This is such a simple idea, I thought somebody must have thought of it before,” Budker said.
He was right. William Happer, a physicist who pioneered spin-polarized spectroscopy and the sodium laser guide stars, had thought of the idea, but had never published it.
“I was very, very happy to hear that, because I felt there may be a flaw in the idea, or that it had already been published,” Budker said.
Researchers map out ice sheets shrinking during Ice Age
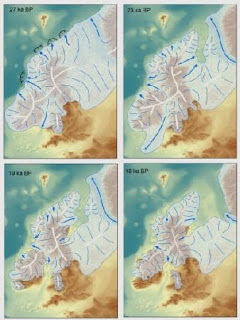
Led by Professor Chris Clark from the University’s Department of Geography, a team of experts developed the maps to understand what effect the current shrinking of ice sheets in parts of the Antarctic and Greenland will have on the speed of sea level rise.
The unique maps record the pattern and speed of shrinkage of the large ice sheet that covered the British Isles during the last Ice Age, approximately 20,000 years ago. The sheet, which subsumed most of Britain, Ireland and the North Sea, had an ice volume sufficient to raise global sea level by around 2.5 metres when it melted.
The maps are based on new information on glacial landforms, such as moraines and drumlins, which were discovered using new technology such as remote sensing data that is able to image the land surface and seafloor at unprecedented resolutions. Experts combined this new information with that from fieldwork, some of it dating back to the nineteenth century, to produce the final maps of retreat.
It is also possible to use the maps to reveal exactly when land became exposed from beneath the ice and was available for colonization and use by plants, animals and humans. This provides the opportunity for viewers to pinpoint when their town/region emerged.
Professor Chris Clark, from the University of Sheffield’s Department of Geography, said: “It took us over 10 years to gather all the information in order to produce these maps, and we are delighted with the results, It is great to be able to visualize the ice sheet and notice that retreat speeds up and slows down, and it is vital of course that we learn exactly why. With such understanding we will be able to better predict ice losses in Greenland and Antarctica.
New model for how Nevada gold deposits formed may help in gold exploration
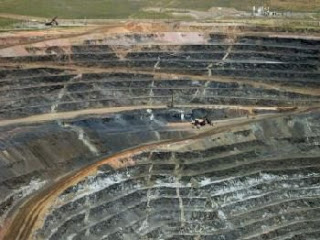
The deposits, known as Carlin-type gold deposits, are characterized by extremely fine-grained nanometer-sized particles of gold adhered to pyrite over large areas that can extend to great depths. More gold has been mined from Carlin-type deposits in Nevada in the last 50 years – more than $200 billion worth at today’s gold prices – than was ever mined from during the California gold rush of the 1800s.
A clearer picture of how rivers and deltas develop
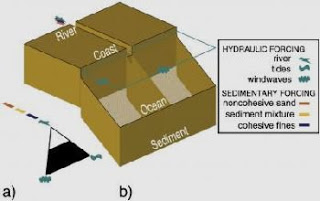
The extra dimension that Geleynse et al. have added to the model is important to delta management, among other things. If – as the Delta Commission recommends – we should be creating “Room for the River”, it is important to know what a river will do with that space. Nathanaël Geleynse explains: “Existing data do not enable us to give ready-made answers to specific management questions … nature is not so easily tamed … but they do offer plausible explanations for the patterns and shapes we see on the surface. The flow system carries the signature of the subsoil, something we were relatively unaware of until now. Our model provides ample scope for further development and for studying various scenarios in the current structure.”
Researcher says the next large central US earthquake may not be in New Madrid
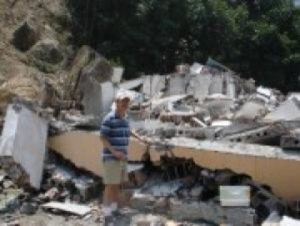
Credit: Image courtesy of University of Missouri-Columbia
This December marks the bicentennial of the New Madrid earthquakes of 1811-12, which are the biggest earthquakes known to have occurred in the central U.S.
Now, based on the earthquake record in China, a University of Missouri researcher says that mid-continent earthquakes tend to move among fault systems, so the next big earthquake in the central U.S. may actually occur someplace else other than along the New Madrid faults.
Mian Liu, professor of geological sciences in the College of Arts and Science at MU, examined records from China, where earthquakes have been recorded and described for the past 2,000 years.
Surprisingly, he found that during this time period big earthquakes have never occurred twice in the same place.
“In North China, where large earthquakes occur relatively frequently, not a single one repeated on the same fault segment in the past two thousand years,” Liu said. “So we need to look at the ‘big picture’ of interacting faults, rather than focusing only on the faults where large earthquakes occurred in the recent past.”
Mid-continent earthquakes, such as the ones that occurred along the New Madrid faults, occur on a complicated system of interacting faults spread throughout a large region.
A large earthquake on one fault can increase the stress on other faults, making some of them more likely to have a major earthquake. The major faults may stay dormant for thousands of years and then wake up to have a short period of activity.
Along with co-authors Seth Stein, a professor of earth and planetary sciences at Northwestern University, and Hui Wang, a Chinese Earthquake Administration researcher, Liu believes this discovery will provide valuable information about the patterns of earthquakes in the central and eastern United States, northwestern Europe, and Australia. The results have been published in the journal Lithosphere.
“The New Madrid faults in the central U.S., for example, had three to four large events during 1811-12, and perhaps a few more in the past thousand years. This led scientists to believe that more were on the way,” Stein said. “However, high-precision Global Positioning System (GPS) measurements in the past two decades have found no significant strain in the New Madrid area.
The China results imply that the major earthquakes at New Madrid may be ending, as the pressure will eventually shift to another fault.”
While this study shows that mid-continent earthquakes seem to be more random than previously thought, the researchers believe it actually helps them better understand these seismic events.
“The rates of earthquake energy released on the major fault zones in North China are complementary,” Wang said. “Increasing seismic energy release on one fault zone was accompanied by decreasing energy on the others. This means that the fault zones are coupled mechanically.”
Studying fault coupling with GPS measurements, earthquake history, and computer simulation will allow the scientists to better understand the mysterious mid-continent earthquakes.
“What we’ve discovered about mid-continent earthquakes won’t make forecasting them any easier, but it should help,” Liu said.
Note: This story has been adapted from a news release issued by the University of Missouri-Columbia
Cave reveals Southwest’s abrupt climate swings during Ice Age
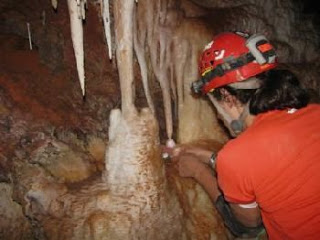
Cole and her colleagues found the Southwest flip-flopped between wet and dry periods during the period studied.
Each climate regime lasted from a few hundred years to more than one thousand years, she said. In many cases, the transition from wet to dry or vice versa took less than 200 years.
“These changes are part of a global pattern of abrupt changes that were first documented in Greenland ice cores,” she said. “No one had documented those changes in the Southwest before.”
Scientists suggest that changes in the northern Atlantic Ocean’s circulation drove the changes in Greenland’s Ice Age climate, Cole said. “Those changes resulted in atmospheric changes that pushed around the Southwest’s climate.”
“Also, changes in the storm track are the kinds of changes we expect to see in a warming world,” she said. “When you warm the North Atlantic, you move the storm track north.”
Cole’s UA co-authors are Jennifer D. M. Wagner, J. Warren Beck, P. Jonathan Patchett and Heidi R. Barnett. Co-author Gideon M. Henderson is from the University of Oxford, U.K.
In such a cave, mineral-rich water percolates through the soil into the cave below and onto its floor. As the water loses carbon dioxide, the mineral known as calcium carbonate is left behind. As the calcium carbonate accumulates in the same spot on the cave floor over thousands of years, it forms a stalagmite.
The researchers chose the particular stalagmite for study because it was deep enough in the cave that the humidity was always high, an important condition for preservation of climate records, Cole said. Following established cave conservation protocols, the researchers removed the formation, which was less than 18 inches tall.
For laboratory analyses, first author Wagner took a core about one inch in diameter from the center of the stalagmite. The scientists then returned the formation to the cave, glued it back into its previous location with special epoxy and capped it with a limestone plug.
To read the climate record preserved in the stalagmite, Wagner sliced the core lengthwise several times for several different analyses.
On one slice, she shaved more than 1,200 hair-thin, 100-micron samples and measured what types of oxygen molecule each one contained.
A rare form of oxygen, oxygen-18, is more common in the calcium carbonate deposited during dry years. By seeing how much oxygen-18 was present in each layer, the scientists could reconstruct the region’s pattern of wet and dry climate.
To assign dates to each wet and dry period, Wagner used another slice of the core for an analysis called uranium-thorium dating.
The radioactive element uranium is present in minute amounts in the water dripping onto a stalagmite. The uranium then becomes part of the formation. Uranium decays into the element thorium at a steady and known rate, so its decay rate can be used to construct a timeline of a stalagmite’s growth.
By matching the stalagmite’s growth timeline with the sequence of wet and dry periods revealed by the oxygen analyses, the researchers could tell in century-by-century detail when the Southwest was wet and when it was dry.
“This work shows the promise of caves to providing climate records for the Southwest. It’s a new kind of climate record for this region,” Cole said.
She and her colleagues are now expanding their efforts by sampling other cave formations in the region.
Earth’s hot past: Prologue to future climate?
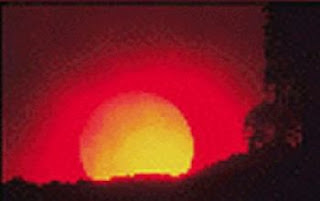
Building on recent research, the study examines the relationship between global temperatures and high levels of carbon dioxide in the atmosphere tens of millions of years ago.
It warns that, if carbon dioxide emissions continue at their current rate through the end of this century, atmospheric concentrations of the greenhouse gas will reach levels that existed about 30 million to 100 million years ago.
Kiehl said that global temperatures may take centuries or millennia to fully adjust in response to the higher carbon dioxide levels.
Accorning to the study and based on recent computer model studies of geochemical processes, elevated levels of carbon dioxide may remain in the atmosphere for tens of thousands of years.
The study also indicates that the planet’s climate system, over long periods of times, may be at least twice as sensitive to carbon dioxide as currently projected by computer models, which have generally focused on shorter-term warming trends.
This is largely because even sophisticated computer models have not yet been able to incorporate critical processes, such as the loss of ice sheets, that take place over centuries or millennia and amplify the initial warming effects of carbon dioxide.
“If we don’t start seriously working toward a reduction of carbon emissions, we are putting our planet on a trajectory that the human species has never experienced,” says Kiehl, a climate scientist who specializes in studying global climate in Earth’s geologic past.
“We will have committed human civilization to living in a different world for multiple generations.”
The Perspectives article pulls together several recent studies that look at various aspects of the climate system, while adding a mathematical approach by Kiehl to estimate average global temperatures in the distant past.
Its analysis of the climate system’s response to elevated levels of carbon dioxide is supported by previous studies that Kiehl cites.
“This research shows that squaring the evidence of environmental change in the geologic record with mathematical models of future climate is crucial,” says David Verardo, Director of NSF’s Paleoclimate Program. “Perhaps Shakespeare’s words that ‘what’s past is prologue’ also apply to climate.”
Kiehl focused on a fundamental question: when was the last time Earth’s atmosphere contained as much carbon dioxide as it may by the end of this century?
If society continues its current pace of increasing the burning of fossil fuels, atmospheric levels of carbon dioxide are expected to reach about 900 to 1,000 parts per million by the end of this century.
Since carbon dioxide is a greenhouse gas that traps heat in Earth’s atmosphere, it is critical for regulating Earth’s climate.
Without carbon dioxide, the planet would freeze over.
This leads to further heating.
Kiehl drew on recently published research that, by analyzing molecular structures in fossilized organic materials, showed that carbon dioxide levels likely reached 900 to 1,000 parts per
million about 35 million years ago.
At that time, temperatures worldwide were substantially warmer than at present, especially in polar regions–even though the Sun’s energy output was slightly weaker.
The high levels of carbon dioxide in the ancient atmosphere kept the tropics at about 9-18 F (5-10 C) above present-day temperatures.
The polar regions were some 27-36 F (15-20 C) above present-day temperatures.
Kiehl applied mathematical formulas to calculate that Earth’s average annual temperature 30 to 40 million years ago was about 88 F (31 C)–substantially higher than the pre-industrial average temperature of about 59 F (15 C).
The study also found that carbon dioxide may have two times or more an effect on global temperatures than currently projected by computer models of global climate.
The world’s leading computer models generally project that a doubling of carbon dioxide in the atmosphere would have a heating impact in the range of 0.5 to 1.0 degrees Celsius watts per square meter. (The unit is a measure of the sensitivity of Earth’s climate to changes in greenhouse gases.)
However, the published data show that the comparable impact of carbon dioxide 35 million years ago amounted to about 2 C watts per square meter.
Computer models successfully capture the short-term effects of increasing carbon dioxide in the atmosphere.
But the record from Earth’s geologic past also encompasses longer-term effects, which accounts for the discrepancy in findings.
The eventual melting of ice sheets, for example, leads to additional heating because exposed dark surfaces of land or water absorb more heat than ice sheets.
“This analysis shows that on longer time scales, our planet may be much more sensitive to greenhouse gases than we thought,” Kiehl says.
Climate scientists are currently adding more sophisticated depictions of ice sheets and other factors to computer models.
As these improvements come on-line, Kiehl believes that the computer models and the paleoclimate record will be in closer agreement, showing that the impacts of carbon dioxide on climate over time will likely be far more substantial than recent research has indicated.
Because carbon dioxide is being pumped into the atmosphere at a rate that has never been experienced, Kiehl could not estimate how long it would take for the planet to fully heat up.
If emissions continue on their current trajectory, “the human species and global ecosystems will be placed in a climate state never before experienced in human history,” the paper states.
Hydrocarbons in the deep Earth?
The scientists ruled out the possibility that catalysts used as part of the experimental apparatus were at work, but they acknowledge that catalysts could be involved in the deep Earth with its mix of compounds.
“We were intrigued by previous experiments and theoretical predictions,” remarked Carnegie’s Alexander Goncharov a coauthor. “Experiments reported some years ago subjected methane to high pressures and temperatures and found that heavier hydrocarbons formed from methane under very similar pressure and temperature conditions. However, the molecules could not be identified and a distribution was likely. We overcame this problem with our improved laser-heating technique where we could cook larger volumes more uniformly. And we found that methane can be produced from ethane.”
Professor Kutcherov, a coauthor, put the finding into context: “The notion that hydrocarbons generated in the mantle migrate into the Earth’s crust and contribute to oil-and-gas reservoirs was promoted in Russia and Ukraine many years ago. The synthesis and stability of the compounds studied here as well as heavier hydrocarbons over the full range of conditions within the Earth’s mantle now need to be explored. In addition, the extent to which this ‘reduced’ carbon survives migration into the crust needs to be established (e.g., without being oxidized to CO2). These and related questions demonstrate the need for a new experimental and theoretical program to study the fate of carbon in the deep Earth.”
Mountain glacier melt to contribute 12 centimeters to world sea-level increases by 2100
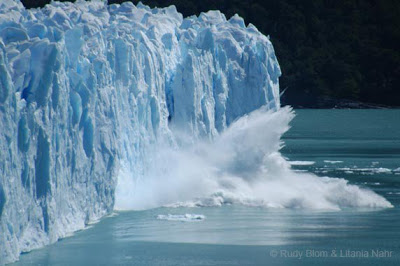
The study modelled volume loss and melt off from 120,000 mountain glaciers and ice caps, and is one of the first to provide detailed projections by region. Currently, melt from smaller mountain glaciers and ice caps is responsible for a disproportionally large portion of sea level increases, even though they contain less than one per cent of all water on Earth bound in glacier ice.
Intergovernmental Panel on Climate Change.
“While the overall sea level increase projections in our study are on par with IPCC studies, our results are more detailed and regionally resolved,” says Radic. “This allows us to get a better picture of projected regional ice volume change and potential impacts on local water supplies, and changes in glacier size distribution.”
Global projections of sea level rises from mountain glacier and ice cap melt from the IPCC range between seven and 17 centimetres by the end of 2100. Radic’s projections are only slightly higher, in the range of seven to 18 centimetres.
Radic’s projections don’t include glacier calving–the production of icebergs. Calving of tide-water glaciers may account for 30 per cent to 40 per cent of their total mass loss.
“Incorporating calving into the models of glacier mass changes on regional and global scale is still a challenge and a major task for future work,” says Radic.
Sulphur proves important in the formation of gold mines
Noble metals, like gold, are transported by magma from deep within the mantle (below the surface) of the Earth to the shallow crust (the surface), where they form deposits. Through a series of experiments, Linnen and his colleagues from the University of Hannover (Germany), the University of Potsdam (Germany) and Laurentian University found that gold-rich magma can be generated in mantle also containing high amounts of sulphur.
Widespread ancient ocean ‘dead zones’ challenged early life
“This work is important at many levels, from the steady growth of atmospheric oxygen in the last 600 million years, to the potential impact of oxygen level fluctuations on early evolution and diversification of life,” said Enriqueta Barrera, program director in the National Science Foundation (NSF)’s Division of Earth Sciences, which funded the research.
Understanding how the environment changed over the course of Earth’s history can give scientists clues to how life evolved and flourished during the critical, very early stages of animal evolution.
“Life and the environment in which it lives are intimately linked,” said Benjamin Gill, the first author of the paper, a biogeochemist at UCR, and currently a postdoctoral researcher at Harvard University.
The team examined the carbon, sulfur and molybdenum contents of rocks they collected from localities in the United States, Sweden, and Australia.
Hot stuff: Magma at shallow depth under Hawaii
“Hawaii was already unique among volcanic systems, because it has such an extensive plumbing system, and the magma that erupts has a unique and variable chemical composition,” Ditkof explained. “Now we know the chamber is at a shallow depth not seen anywhere else in the world.”
Ancient raindrops reveal a wave of mountains sent south by sinking Farallon plate
“We saw a major isotopic shift at around 49 million years ago, in southwest Montana,” he said. “And another one at 39 mya, in northern Nevada” as the uplift moved southward. Previous work by Chamberlain’s group had found evidence for these shifts in data from two basins, but Mix’s work with the larger data set demonstrated that the pattern of uplift held across the entire western U.S.
“The peeling plate looked sort of like a tongue curling down,” said Page Chamberlain, a professor in environmental Earth system science who is Mix’s advisor.
“There was a big north to south sweep of volcanism through the western U.S. at the exact same time,” he said.
“The pattern of topographic uplift we found matches what has been documented by other people in terms of the volcanology and extension,” Mix said.
“Those three things together, those patterns, all point to something going on with the Farallon plate as being responsible for the construction of the western mountain ranges, the Cordillera.”
“The main implication of this work is that it was not a plateau that collapsed, but rather something that happened in the mantle, that was causing this mountain growth,” Chamberlain said.
First measurement of magnetic field in Earth’s core
The magnetic field strength is 25 Gauss, or 50 times stronger than the magnetic field at the surface that makes compass needles align north-south. Though this number is in the middle of the range geophysicists predict, it puts constraints on the identity of the heat sources in the core that keep the internal dynamo running to maintain this magnetic field
“This is the first really good number we’ve had based on observations, not inference,” said author Bruce A. Buffett, professor of earth and planetary science at UC Berkeley. “The result is not controversial, but it does rule out a very weak magnetic field and argues against a very strong field.”
“A measurement of the magnetic field tells us what the energy requirements are and what the sources of heat are,” Buffett said.
“You get changes in the surface magnetic field that look a lot like gyres and flows in the oceans and the atmosphere, but these are being driven by fluid flow in the outer core,” Buffett said.
“The moon is continually forcing the rotation axis of the core to precess, and we’re looking at the response of the fluid outer core to the precession of the inner core,” he said.
New way found of monitoring volcanic ash cloud
As the researchers write, “When a plume becomes sufficiently electrified to produce lightning, the rate of lightning generation provides a method of remotely monitoring the plume height, offering clear benefits to the volcanic monitoring community.”
Using chaos to model geophysical phenomena
“Nevertheless, it is very important that scientists can quantify the ‘transport’ properties of these geophysical systems: Put very simply, how does a packet of air or water get from A to B, and how large are these packets? An example of one of these packets is the Antarctic polar vortex, a rotating mass of air in the stratosphere above Antarctica that traps chemicals such as ozone and chlorofluorocarbons (CFCs), exacerbating the effect of the CFCs on the ozone hole,” Froyland says.
New research shows rivers cut deep notches in the Alps’ broad glacial valleys
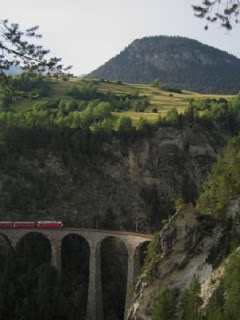
“The alpine inner gorges appear to lay low and endure glacial attack. They are topographic survivors,” Montgomery said.
“The answer is not so simple that the glaciers always win. The river valleys can hide under the glaciers and when the glaciers melt the rivers can go back to work.”
“That means the glaciers aren’t cutting down the bedrock as fast as the rivers do. If the glaciers were keeping up, each time they’d be able to erase the notch left by the river,” Montgomery said.
SCEC’s ‘M8’ earthquake simulation breaks computational records, promises better quake models

The “M8” simulation represents how a magnitude 8.0 earthquake on the southern San Andreas Fault will shake a larger area, in greater detail, than previously possible. Perhaps most importantly, the development of the M8 simulation advances the state-of-the-art in terms of the speed and efficiency at which such calculations can be performed.