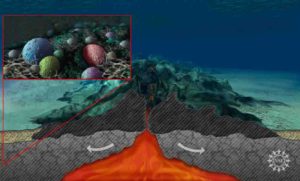
Just about all life on Earth — from the jumbo-jet-sized blue whale to tiny microbes — use carbon in one form or another.
In the deep ocean, though, all carbon is not created equal.
While some, like sugars and proteins, is quickly gobbled up by the micro-organisms that call the ocean home, some — the chitin found in fish scales and marine exoskeletons — is far harder to consume. Scientists have long believed relatively little of that so-called “refractory carbon” is eaten. Much of it simply falls to the ocean floor and helps make up deep-water sediment, although some small refractory molecules stay suspended in the ocean and float along with currents for thousands of years.
But a team of researchers, led by Professor of Organismic and Evolutionary Biology Peter Girguis and Suni Shah Walter, then a post-doctoral fellow in Girguis’ lab, has shown that underground aquifers along the mid-ocean ridge act like natural biological reactors, pulling in cold, oxygenated seawater, and allowing microbes to break down more — perhaps much more — refractory carbon than scientists ever believed. The study is described in an April 23 paper published in Nature Geoscience.
“This has the real prospect of reshaping the way we think about carbon cycling in the deep ocean,” Girguis said. “Instead of setting up a tiny reactor here in the lab with maybe ten liters of water and pumping it around to get some result, this is a natural lab.
“At the end of the day our community wants to know what happens to carbon,” he continued. “We know it is produced at the surface by photosynthetic algae. We know fish eat some of it. We know some of it sinks. We can account for that, but we have some giant holes in our budget.”
While the study begins to close those gaps, it also helps illuminate a part of the deep-ocean carbon cycle that had been a mystery.
“We don’t know where all of that old carbon goes, and underground aquifers are part of the answer,” Shah Walter added. “The majority of fluids that circulate through the crust might look like this, we just didn’t know much about what was going on in them before.”
“This work shows that the vast subseafloor community of microbes could be fed by seawater circulating through deep ocean crust,” said Michael Sieracki, program director in the National Science Foundation’s Division of Ocean Sciences, which funded the research. “In turn, these microbes change the composition of the seawater that then circulates back into the ocean.”
At the heart of the system, Girguis said, is the mid-ocean ridge, a massive chain of undersea mountains that circle the globe.
A key part in the development of the theory of tectonics, in the deep ocean the ridge system acts like a convection cell — water seeps into cracks and fissures on either side of the ridge and is heated as it gets closer to the axis, where magma is close to the surface. As that heated water rises, cold, oxygenated seawater is pulled into the rocks, creating a massive undersea aquifer.
For scientists — like Girguis — working to understand ocean carbon cycles, the aquifer represents a rare chance to study the ocean under virtually ideal conditions.
“Our understanding of where carbon goes in the ocean is pretty rudimentary,” he said. “This gives us an opportunity to go to this natural laboratory and for the first time…take a measurement at point A and a measurement at point B and talk about what happens between them. That’s a rare opportunity in the deep sea. There is nowhere in the deep ocean that we can do that in the water column — it’s impossible because it’s too well-mixed.”
To get those measurements, Girguis and colleagues targeted a site in the mid-Atlantic they dubbed the “North Pond.”
In 2011, an international team of researchers drilled a series of wells into the seafloor at the site and collected water samples to identify which microbes call the aquifer home and whether they were capable of consuming carbon.
“We wanted to tap into this and collect pristine water and microbial samples,” he said. “When you look at these mid-ocean ridge systems and think about the water that is circulating through them, it looks as though a lot of the ocean is circulating through this cold, oxic environment…we wanted to know what’s going on there with the microbes and what’s happening with the carbon they get.”
“Why do we care?” he continued. “We care about the fate of carbon because the carbon cycle is extremely important, not just for climate change, but for helping us to understand how the oceans work.”
But when the team began collecting samples from the aquifer, Shah Walter said, they were in for a surprise.
“When the team pulled the fluids up from the aquifer, they didn’t expect it to be as similar to seawater as it was,” Shah Walter said. “All the planning was for fluids that looked more like other hydrothermal fluids (which can show high levels of methane and lower levels of oxygen.) They were prepared to measure methane, for example. But at first glance North Pond fluids looked chemically very similar to seawater, so that’s when I got pulled in, because my work has focused on the open ocean. So the fact that this was a unique environment, that we could use this natural incubator, that became clear to us after the samples came up.”
While Girguis and colleagues were able to show microbes in the aquifer could eat carbon, it remained unclear how active they were.
“With the limited data we have, we have been able to show that water comes in with a certain oxygen concentration, and as it drops, the carbon goes down in direct proportion,” he said. “That gives us a high degree of confidence that microbes are eating it and using oxygen.”
In fact, Girguis said, it’s possible that microbes are eating carbon at an impressive rate.
“The number we found was that about 50 percent of the carbon is consumed, but it’s important to realize that number is very conservative,” he said. “If you consider how little we know about this system…it begs the question of what happens to this water as it continues to flow through here. It’s not hard to imagine that we might eventually find that the majority of the carbon being eaten.”
In later tests, Girguis and colleagues showed as water moves through the aquifer over nearly 3,000 years, the easy-to-eat carbon is consumed quickly — within just a few hundred years. More importantly, they also found signs that refractory carbon was also being eaten.
“What’s exciting is that for a long time — and even today — there have been debates about how quickly (refractory carbon) can be eaten,” Girguis said. “But this natural laboratory has showed us that not only is this being degraded biologically, but the time over which it’s being eaten.”
Ultimately, Girguis said, the study highlights the way in which understanding the deep ocean can lead to a better overall understanding of the ocean.
“We think that the entire ocean circulates through this mid-ocean ridge system every 100,000 to 200,000 years,” Girguis said. “If the entire ocean is circulating through this aquifer, we think this is probably the best representation of a typical microbial-seawater interaction as it moves through that system.
“Until we had these data there was a big gap in our knowledge,” he added. “We thought water gets pulled in here, and all the action happens when it gets hot. But we don’t think that’s true anymore, especially because these microbes are eating this difficult-to-eat carbon.”
Going forward, Girguis and colleagues hope to better quantify how much carbon is consumed as seawater moves through the aquifer, and whether the minerals in the basalt rock may contribute to microbe’s ability to consume refractory carbon.
Ongoing studies, Shah Walter said, are testing the aquifer in other locations, and to test whether microbes are also consuming particulate matter in the aquifer.
“The majority of fluid that circulates through the crust is cool like this, and so far this is the only place we’re looking at, so we definitely need to look in other spots,” she said. “But there is also still a hole in our carbon budget. We have an idea that one missing piece of the puzzle is that we weren’t looking at the particles in the water. We think we can close this hole by accounting for the carbon metabolism that’s happening on those particles.”
“This started with the intent of saying…let’s figure out what the microbes are doing in this cold, oxygenated aquifer, and we can add those data to our understanding of the carbon cycle,” Girguis said. “But in looking at the data, we were rather astonished by the results, which tell us that, not surprisingly, this easy-to-eat carbon is consumed first, but then we began to see signs of the consumption of this refractory carbon.
“That is a big deal,” he added. “One way to think about this is that all creatures depend on carbon, and there’s this cycle where carbon is produced and consumed…but studying that in the water column is impractical. But take that water and pump it through an underground, oxygenated aquifer and you suddenly have an opportunity to take a closer look at these processes.”
This research was supported with funding from the German Science Foundation, the National Science Foundation, the WHOI Postdoctoral Scholar Program and the NSF Cooperative Agreement for the Operation of a NOSAMS Facility, The Center for Dark Energy Biosphere Investigations and The Gordon and Betty Moore Foundation.
Reference:
Sunita R. Shah Walter, Ulrike Jaekel, Helena Osterholz, Andrew T. Fisher, Julie A. Huber, Ann Pearson, Thorsten Dittmar, Peter R. Girguis. Microbial decomposition of marine dissolved organic matter in cool oceanic crust. Nature Geoscience, 2018; DOI: 10.1038/s41561-018-0109-5
Note: The above post is reprinted from materials provided by Harvard University.